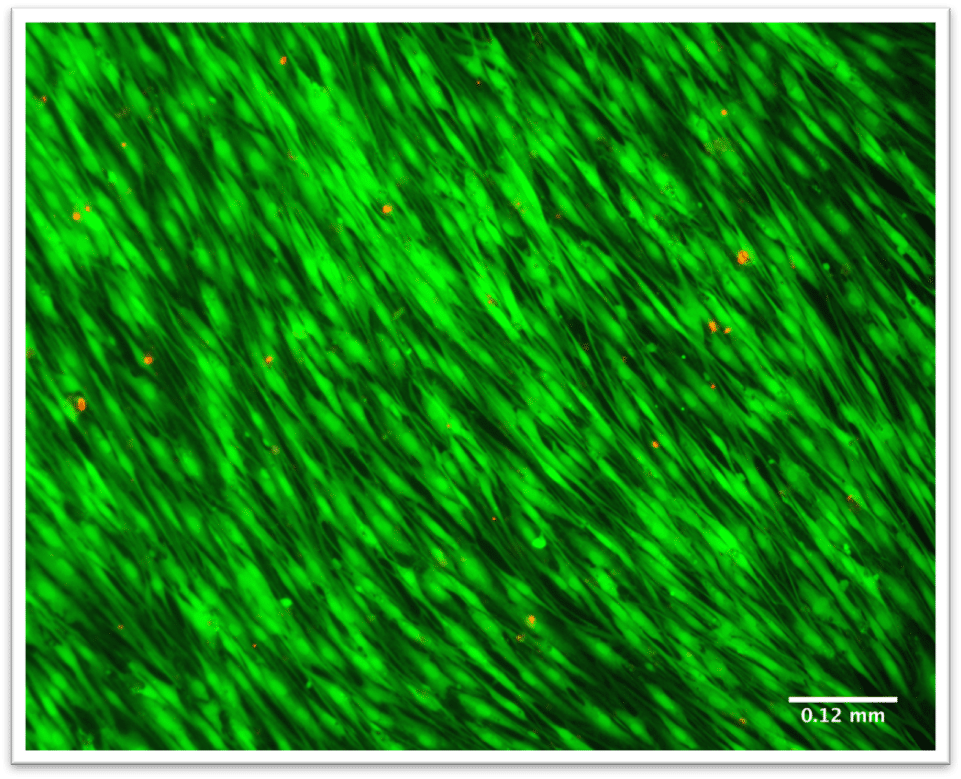
To choose the right cell density for bioprinting, you first need to consider your application and the final assays you would like to perform. This article will help you decide on the cellular density for your bioink.
Cell Density in vivo
Each organ in the body has a specific cellular density, however accurate estimation of this value is not easy. There are different approaches based on immunocytochemistry, DNA content measurement or mathematical calculations [1]. For example, the liver is estimated to contain 1.3×108 cells in 1 gram of tissue [2]. This is dramatically different than the average cell densities for in vitro cell culture of around 1×105 cells/cm2 [3]. What’s more, tissue density is dynamic and may change during the development of the organism as well as during pathogenic processes.
Cell Density in vitro
The number of cells initially seeded in a given in vitro system can have a great impact on the culture outcome. Density-depended regulation of gene expression, differentiation, proliferation, and apoptosis are key elements in this equation. Particular parameters will vary depending on a cell type. For example, cancer cells are known to lack a contact inhibition mechanism allowing them to grow in relatively high densities without reduction of proliferation [4,5]. The density of the cell culture can also influence the accumulation of drugs, resulting in different responses to compounds tested [6]. Given all these facts, cell density should be optimized and selected to mimic the native tissue functionally and physiologically.
Biological Considerations
To begin, ask yourself these questions:
- What is the native composition of the tissue you are trying to mimic?
- Are the cells you are using proliferating or are they post-mitotic?
- How long are you planning to keep the construct in the cell culture?
Starting too low with the initial cell number might inhibit cell growth due to lack of cell-cell interaction or limited growth factor secretion and thus insufficient cell-signaling stimulation. If the construct is designed to be transplanted in vivo, cell number is also an important parameter. Not enough cells in the bioink can lead to poor integration with host tissue [8].
On the other hand, starting with too many cells can result in a quick contact inhibition of the proliferation, overgrowing of cell clusters resulting in the development of hypoxic conditions, limited nutrient flow, or rapid nutrient consumption with significant waste build-up. The last consideration is especially important because media volumes often used for 2D culture are not sufficient to support the growth of high-density 3D cell constructs. Thus, if your media contains phenol-red and you observe a rapid change of media color, it might indicate that the culture media volume should be increased, or media change should be more frequent [7,8]. High cell concentration can also have a positive effect on ECM production, hydrogel remodeling and creating the right environment for the cells [9].
Mechanical Considerations
Adding a cellular component to your biomaterial will change its properties. High cell density will increase the viscosity of the hydrogel in which cells are suspended [7]. A higher-viscosity bioink will require increased pressure for dispensing, which can negatively impact cell viability [this, however, could be compensated with larger nozzle diameter]. High cellular content can also decrease hydrogel stiffness [9]. These factors will influence the geometry of the deposited strand and its mechanical strength, so if high resolution and long term maintenance of the construct are required, printing parameters should be optimized in respect to the cellular density of the bioink [10].
Cell Number Optimization
To optimize cell number for your application, you should start with a range of different densities. You can find the cell number used by some Allevi authors in their publications in Table 1 below. It is a great starting point if you are planning to use a similar combination of cells and biomaterials.
For the best results, run simple biocompatibility studies including several concentrations of cells in your bioink. Start by looking into initial and endpoint cell viability and proliferation. Later, you can check for functional outcomes in more detail. This will depend on your experiments but think about the end-goal and what your construct should reflect: correct drug response, following in vivo development, or expressing some desired markers. For the final bioprint, remember to adjust your printing conditions to maintain high cell viability and resolution.
Cell type | Bioink | Cell density | Reference number |
---|---|---|---|
Human dermal fibroblasts [CRL-2522, ATCC] | GelMa | 3×106/ml | 11 |
Neural Progenitor Cells | Alginate | 3×107/ml | 12 |
Breast cancer cells [MCF-7] | GelMa | 1×106/ml 5×106/ml 1×107/ml 5×107/ml | 13 |
Human primary knee articular chondrocytes [hKAC] | Collagen I/Agarose | 2×106/ml | 14 |
Patient specific appendiceal cancer cells | HA/collagen | 1 x107/ml | 15 |
Human hepatic stellate cell line [L X 2] Primary fetal activated hepatic stellate cells [aHSC] | Methacrylated COL I/Thiolated HA | 5×106/ml | 16 |
Primary human neonatal dermal fibroblasts hiPSC-derived ventral sNPC miPSC-derived OPCs | Matrigel Gelatin/fibrin GelMa | 1×107/ml | 17 |
Human adipose tissue-derived mesenchymal stem cells [hAD-MSCs] | GelMa | 1.5×106/ml | 18 |
Cardiac myocytes Cardiac fibroblasts | GelMA | 1×106/ml | 19 |
Neuroblastoma [SKN-BE-2] | Sodium alginate | 1×107/ml | 10 |
hMSCs | MeAlg MeHA | 2×106/ml | 20 |
[1] E. Bianconi et al., An estimation of the number of cells in the human body, Ann. Hum. Biol. 40 (2013) 463–471.
[2] R. Marcos, R.A.F. Monteiro, E. Rocha, Design-based stereological estimation of hepatocyte number, by combining the smooth optical fractionator and immunocytochemistry with anti-carcinoembryonic antigen polyclonal antibodies, Liver Int. 26 (2006) 116–124.
[3] R.S.D.L.B.S.E.D.M.E.K.I.N.M. J. Saragih, ATCC ® ANIMAL CELL CULTURE GUIDE tips and techniques for continuous cell lines, 2011.
[4] B.K. Gage, T.D. Webber, T.J. Kieffer, Initial Cell Seeding Density Influences Pancreatic Endocrine Development During in vitro Differentiation of Human Embryonic Stem Cells, PLoS One. 8 (2013) e82076.
[5] O. V. Leontieva, Z.N. Demidenko, M. V. Blagosklonny, Contact inhibition and high cell density deactivate the mammalian target of rapamycin pathway, thus suppressing the senescence program, Proc. Natl. Acad. Sci. U. S. A. 111 (2014) 8832–8837.
[6] Y. Takemura et al., The influence of tumor cell density on cellular accumulation of doxorubicin or cisplatin in vitro, Cancer Chemother. Pharmacol. 27 (1991) 417–422.
[7] N. Cao, X.B. Chen, D.J. Schreyer, Influence of Calcium Ions on Cell Survival and Proliferation in the Context of an Alginate Hydrogel, ISRN Chem. Eng. 2012 (2012) 1–9.
[8] G. Cidonio, M. Glinka, J.I. Dawson, R.O.C. Oreffo, The cell in the ink: Improving biofabrication by printing stem cells for skeletal regenerative medicine, Biomaterials. 209 (2019) 10–24.
[9] K. Hölzl et al., Bioink properties before, during and after 3D bioprinting, Biofabrication. 8 (2016) 032002.
[10] J. Lewicki, J. Bergman, C. Kerins, O. Hermanson, Optimization of 3D bioprinting of human neuroblastoma cells using sodium alginate hydrogel, Bioprinting. 16 (2019) e00053.
[11] Y.S. Zhang et al., Bioprinted thrombosis-on-a-chip, Lab Chip. 16 (2016) 4097–4105.
[12] C.D. Lindsay, J.G. Roth, B.L. LeSavage, S.C. Heilshorn, Bioprinting of stem cell expansion lattices, Acta Biomater. (2019).
[13] X. Cao et al., A Tumor-on-a-Chip System with Bioprinted Blood and Lymphatic Vessel Pair, Adv. Funct. Mater. 29 (2019) 1807173.
[14] D.F.D. Campos et al., Synchronized Dual Bioprinting of Bioinks and Biomaterial Inks as a Translational Strategy for Cartilage Tissue Engineering, 3D Print. Addit. Manuf. 6 (2019) 63–71.
[15] K.I. Votanopoulos et al., Appendiceal Cancer Patient-Specific Tumor Organoid Model for Predicting Chemotherapy Efficacy Prior to Initiation of Treatment: A Feasibility Study, Ann. Surg. Oncol. 26 (2019) 139–147.
[16] A. Mazzocchi et al., Optimization of collagen type I-hyaluronan hybrid bioink for 3D bioprinted liver microenvironments, Biofabrication. 11 (2019) 015003.
[17] D. Joung et al., 3D Printed Stem-Cell Derived Neural Progenitors Generate Spinal Cord Scaffolds, Adv. Funct. Mater. 28 (2018) 1–10.
[18] I. Pepelanova, K. Kruppa, T. Scheper, A. Lavrentieva, Gelatin-methacryloyl (GelMA) hydrogels with defined degree of functionalization as a versatile toolkit for 3D cell culture and extrusion bioprinting, Bioengineering. 5 (2018).
[19] P. Koti et al., Use of GelMA for 3D printing of cardiac myocytes and fibroblasts, J. 3D Print. Med. 3 (2019) 11–22
[20] S. Ji, E. Almeida, M. Guvendiren, 3D bioprinting of complex channels within cell-laden hydrogels, Acta Materialia Inc., 2019.